Unit 02
Unit 02
Unit 02
Unit 02
Q-Discuss electromagnetic radiation.
Electromagnetic radiation refers to the waves of the electromagnetic field, propagating through space, carrying electromagnetic radiant energy. It includes radio waves, microwaves, infrared, ultraviolet, X-rays, and gamma rays.
Water waves transmit energy through space by the periodic oscillation of matter. In contrast, energy that is transmitted, or radiated, through space in the form
Of periodic oscillations of electric and magnetic fields is known as electromagnetic radiation, which is energy that is transmitted through space in the form of periodic oscillations of electric and magnetic fields. Some forms of electromagnetic radiation. In a vacuum, all forms of electromagnetic radiation whether microwaves, visible light, or gamma rays travel at the speed of light (c), which is the speed with which all forms of electromagnetic radiation travel in a vacuum, a fundamental physical constant with a value of 2.99792458 × 108 m/s. This is about a million times faster than the speed of sound. The energy of electromagnetic radiation is directly proportional to its frequency and inversely proportional to its wavelength:
E∝u
E∝1/λ
Q-Explain spectrophotometer.
A rainbow is a most beautiful thing which can be seen, but if it was not for light then how it behaves, we wouldn't be able to see it. Raindrops act like a prism, splitting the combined white light from the sun into all the visible colors of the light spectrum.
But even the colors of simple things, like a leaf for example; have to do with wavelengths of light. Each color in light has a different wavelength, so when light reaches an object, some wavelengths get absorbed, and others get reflected back. We only see the reflected colors. A green leaf only appears green because all the other colors have been absorbed.
It is this same principle of color and wavelength that a spectrophotometer is based on. A spectrophotometer is a special instrument that measures how much light a substance absorbs. Every substance will reflect back and absorb light slightly differently. Like how a fingerprint identifies each individual human, knowing exactly how much red or green, or blue, etc. gets absorbed allows us to identify and quantify different materials.
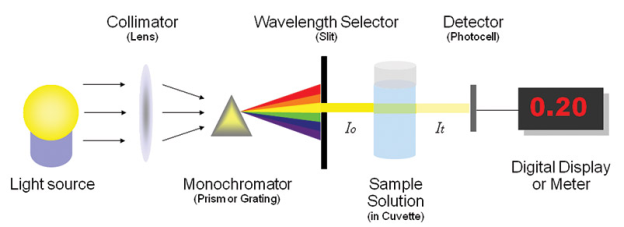
Q-What is monochromator? Explain its application.
Monochromator used to measure the wavelength of the light. Monochromator is placed between the sample and the source of light passing from that the light passes to the detector. Here the monochromatic used to detect the wavelength of the light form the source. While on moving further there is a Double beam Ultraviolet-Visible instrument is used here is a splitter and a series of mirrors to get the beam to a reference sample and the sample to be analyzed, this allows for more accurate readings. In the simultaneous Ultraviolet-Visible instruments monochromator is removed hence it has diode array detectors that allow the instrument to simultaneously detect the absorbance at all wavelengths. This instrument is much faster and accurate than double and single Ultraviolet-Visible instrument.
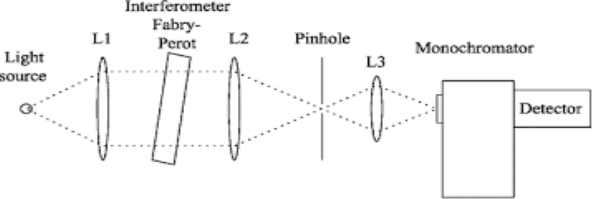
Applications:
1- This is majorly used in the analytical chemistry.
2- This can be used as a detector for the High performance liquid chromatography.
Ultraviolet-Visible Spectroscopy is used as the semiconductor in the industry to measure the thickness and optical properties of wafer.
Q-What is chromophore?
It is used in the denotation of some structural features of which gives color to compound. E.g. Nitro group is a chromophore because its presence in a compound gives yellow color to the compound. But these days the term chromophore is used in a much broader sense which may be defined as “any group which exhibit absorption of electromagnetic radiation in a visible or ultra-visible region “It may or may not impart any color to the compound. Some of the important chromophores are: ethylene, acetylene, carbonyls, acids, esters and nitrile groups etc. There are two types of chromophores:
1. Chromophores in which the groups have π electrons undergo π-π* transitions. For examples:-ethylenes, acetylenes etc.
2. Chromophores having both π- electrons and n (non-bonding) electrons undergo two types of transitions. i.e., π-π* and n-π*, for examples: - carbonyls, nitriles and nitro compounds etc.
Q-What is auxochromophores?
It is a group which itself does not act as a chromophore but when attached to a chromophore, it shifts the adsorption towards longer wavelength along with an increase in the intensity of absorption. Some commonly known auxochromic groups are: -OH, -NH2, -OR, -NHR, and –NR2. For example: When the auxochrome –NH2 group is attached to benzene ring. Its absorption change from λ max 225 (ɛmax 203) to λmax 280 (εmax1430)
All auxochromes have one or more non-bonding pairs of electrons. If an auxochromes is attached to a chromophore, it helps is extending the conjugation by sharing of non-bonding pair of electrons as shown below.
CH2 = CH – NR2 ------------- > CH2-CH-NH2
The extended conjugation has been responsible for bath chromic effect of auxochromes.
Q-Explain electronic excitation in conjugated dienes.
Ethene absorbs light, it undergoes a π - π* transition. Because π- π* energy gaps are narrower than σ - σ* gaps, ethene absorbs light at 165 nm a longer wavelength than molecular hydrogen.
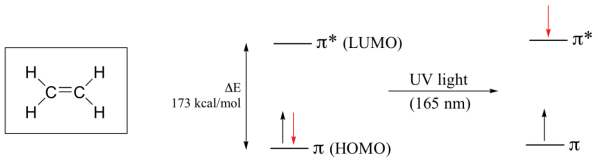
The electronic transitions of both molecular hydrogen and ethene are too energetic to be accurately recorded by standard UV spectrophotometers, which generally have a range of 220 – 700 nm. Where UV spectroscopy becomes useful to most organic and biological chemists is in the study of molecules with conjugated pi systems. In these groups, the energy gap for π -π* transitions is smaller than for isolated double bonds, and thus the wavelength absorbed is longer. Molecules or parts of molecules that absorb light strongly in the UV-vis region are called chromophores.
Next, we'll consider the 1,3-butadiene molecule. From valence orbital theory alone we might expect that the C2-C3 bond in this molecule, because it is a sigma bond, would be able to rotate freely.
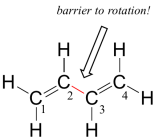
Experimentally, however, it is observed that there is a significant barrier to rotation about the C2-C3 bond, and that the entire molecule is planar. In addition, the C2-C3 bond is 148 pm long, shorter than a typical carbon-carbon single bond (about 154 pm), though longer than a typical double bond (about 134 pm).
Molecular orbital theory accounts for these observations with the concept of delocalized π bonds. In this picture, the four p atomic orbitals combine mathematically to form four pi molecular orbitals of increasing energy. Two of these - the bonding pi orbitals - are lower in energy than the p atomic orbitals from which they are formed, while two - the antibonding pi orbitals - are higher in energy.
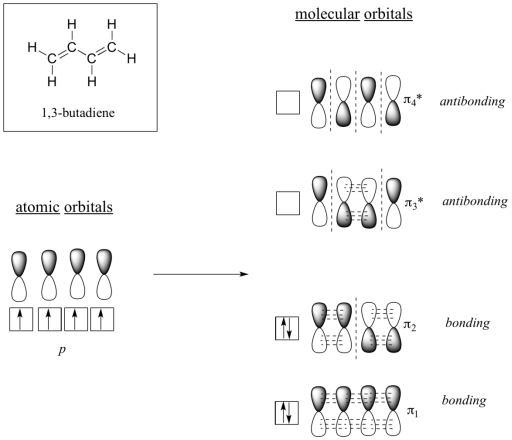
The lowest energy molecular orbital, pi1, has only constructive interaction and zero nodes. Higher in energy, but still lower than the isolated p orbitals, the pi2 orbital has one node but two constructive interactions - thus it is still a bonding orbital overall. Looking at the two antibonding orbitals, pi3* has two nodes and one constructive interaction, while pi4* has three nodes and zero constructive interactions.
By the aufbau principle, the four electrons from the isolated 2pz atomic orbital are placed in the bonding pi1 and pi2 MO. Because pi1 includes constructive interaction between C2 and C3, there is a degree, in the 1, 3-butadiene molecules, of pi-bonding interaction between these two carbons, which accounts for its shorter length and the barrier to rotation. The valence bond picture of 1,3-butadiene shows the two pi bonds as being isolated from one another, with each pair of pi electrons ‘stuck’ in its own pi bond. However, molecular orbital theory predicts (accurately) that the four pi electrons are to some extent delocalized, or ‘spread out’, over the whole pi system.
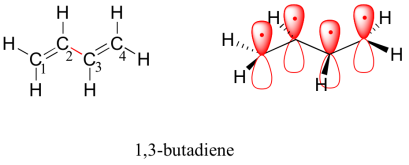
1, 3-butadiene is the simplest example of a system of conjugated pi bonds. To be considered conjugated, two or more pi bonds must be separated by only one single bond – in other words, there cannot be an intervening sp3-hybridized carbon, because this would break up the overlapping system of parallel p orbital. In the compound below, E.g, the C1-C2 and C3-C4 double bonds are conjugated, while the C6-C7 double bond is isolated from the other two pi bonds by sp3-hybridized C5.
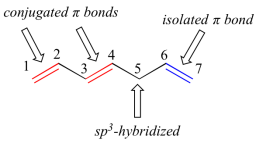
A very important concept to keep in mind is that there is an inherent thermodynamic stability associated with conjugation. This stability can be measured experimentally by comparing the heat of hydrogenation of two different dines. When the two conjugated double bonds of 1,3-pentadiene are 'hydrogenated' to produce pentane, about 225 kJ is released per mole of pentane formed. Compare that to the approximately 250 kJ/mol released when the two isolated double bonds in 1,4-pentadiene are hydrogenated, also forming pentane.
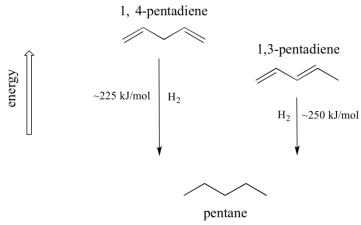
The conjugated diene is lower in energy: in other words, it is more stable. In general, conjugated pi bonds are more stable than isolated pi bonds.
Q-What is Beer Lambart’s law? Derive it.
The amount of energy transmitted or absorbed by a solution is proportional to the solutions molar absorptive and the concentration of solute. In simple terms, a more concentrated solution absorbs more light than a more dilute solution does. Mathematically this can be expressed by
A=ε/C
Where,
A = absorption,
Ε = molar attenuation coefficient
I = path length
C = concentration of the solution
Derivation of the Beer-Lambert law
The Beer-Lambert law can be derived from an approximation for the absorption coefficient for a molecule by approximating the molecule by an opaque disk whose cross-sectional area represents the effective area seen by a photon of frequency w. If the frequency of the light is far from resonance, the area is approximately 0, and if w is close to resonance the area is a maximum. Taking an infinitesimal slab, dz, of sample:
Io is the intensity entering the sample at z=0, Iz is the intensity entering the infinitesimal slab at z, dI is the intensity absorbed in the slab, and I is the intensity of light leaving the sample. Then, the total opaque area on the slab due to the absorbers is * N * A * dz. Then, the fraction of photons absorbed will be
* N * A * dz / A so,
DI / Iz = - * N * dz
Integrating this equation from z = 0 to z = b gives:
In (I) – ln (Io) = - * N * b
- ln (I / Io) = * N * b.
Since N (molecules/cm3) * (1 mole / 6.023x1023 molecules) * 1000 cm3 / liter = c (moles/liter) and 2.303 * log(x) = ln(x) then
- log (I / Io) = * (6.023x1020 / 2.303) * c * b
- log (I / Io) = A = * b * c
Where,
- =
* (6.023x1020 / 2.303) =
* 2.61x1020
Q-Explain selection rule and applications of vibrational spectroscopy.
On the irradiation of the sample with infrared electromagnetic radiation then its frequency can be measured by the Infrared spectroscopy. It is a technique used to study the vibrations between atoms because atomic vibrational excitations occur in the infrared region of the electromagnetic spectrum. The bonds between atoms can be thought of as a spring connecting two masses. In the spring-mass analogy the moving system can be approximated by a simple harmonic oscillator. The frequency oscillation is proportional to
√k/m
Where,
k is the spring constant
m is the mass of the object.
Applications:
1-Infrared technique is used in organic and inorganic chemistry.
2-This is used in quality control, dynamic measurement.
3-This is majorly used in the forensic labs.
4-Infrared Spectroscopy is used in measuring the degree of polymerization in manufacturing the polymers.
Q-What is NMR? Explain.
NMR stands for the Nuclear Magnetic Resonance Spectroscopy. NMR Spectroscopy technique is used to observe local magnetic fields around atomic nuclei. An NMR instrument allows the molecular structure of a material to be analyzed by observing and measuring the interaction of nuclear spins when placed in a powerful magnetic field. For the analysis of molecular structure at the atomic level, electron microscopes and X-ray diffraction instruments can also be used, but the advantages of NMR are that sample measurements are non-destructive and there is less sample preparation required.
Nuclear magnetic resonance (NMR) spectroscopy is a crucial analytical tool for organic chemists. The research in the organic lab has been significantly improved with the aid of the NMR. Not only can it provide information on the structure of the molecule, it can also determine the content and purity of the sample. Proton (1H) NMR is one of the most widely used NMR methods by organic chemists. The protons present in the molecule will behave differently depending on the surrounding chemical environment, making it possible to elucidate their structure.
Chemical shift in NMR spectroscopy:
A spinning charge generates a magnetic field that results in a magnetic moment proportional to the spin. In the presence of an external magnetic field, two spin states exist; one spin up and one spin down, where one aligns with the magnetic field and the other opposes it.
Chemical shift is characterized as the difference between the resonant frequency of the spinning protons and the signal of the reference molecule. Nuclear magnetic resonance chemical change is one of the most important properties usable for molecular structure determination. There are also different nuclei that can be detected by NMR spectroscopy, 1H (proton), 13C (carbon 13), 15N (nitrogen 15), 19F (fluorine 19), among many more. 1H and 13C are the most widely used. The definition of 1H as it is very descriptive of the spectroscopy of the NMR. Both the nuts have a good charge and are constantly revolving like a cloud. Through mechanics, we learn that a charge in motion produces a magnetic field. In NMR, when we reach the radio frequency (Rf) radiation nucleus, it causes the nucleus and its magnetic field to turn (or it causes the nuclear magnet to pulse, thus the term NMR).
Q-Write down the applications of NMR.
- It is non-destructive. That means it does not damage/destroy your sample and you can use that sample to do other experiments.
- You can use both solid and liquid phases on it. Whereas some instruments are limited to only the solid or liquid phase, NMR has both. The advantage to this is not everything can be crystallized, so being able to determine various properties of the molecule in liquid phase is very important.
- There are a large amount of things we can observe using NMR, and that is growing even more. We can work with very small and very large molecules with NMR. We can observe various conformations, dynamics, structure, and more at a single-molecule level.
- Not only can we use it for a large variety of molecular sizes, but we can also use it for a variety of temperatures (from 77K to over 350K).
- We can also observe in-cell processes happening in real time too.